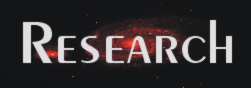 |
Welcome to the research section of my homepage!
I'm a Professor and Canada Research Chair (Tier II) at Saint
Mary's
University
(formerly Queen's University, Mcmaster University, and U.C. Berkeley)
working on
large scale structure and galaxy formation. I also have links with the
Virgo,
Hydra
and Seattle
Consortia. My work is focused primarily on using
simulations to aid our understanding of the structure formation process.
I also have a 304-core computer cluster dedicated to my research group
that enables to perform simulations that would not normally be possible
in a shared queue environment.
You can link to a list of my publications
and presentations .
Former and current students are listed on my "friends" page.
2016 Grad student research opportunities
I am very interested in taking on
new
students, both at
the MSc and
PhD level.
Although I encourage
students to think
independently and
come up with research projects that are mutually agreeable, I currently
have particular interest in the following student projects:
(1) Development of the next generation of parallel
cosmological
simulation codes (ideally at the PhD level) and large-scale
simulations. I've recently starting working with the GIZMO code developed
by Phil Hopkins at Caltech and I'm looking to add a number of revised
feedback routines to the code.
(2) Ongoing studies into the representation of supernova feedback
in galaxy formation, and addressing the "bulge" issue in current
numerical simulations of galaxy formation. This project may also tie in
closely with observations of disk galaxies.
(3) Connecting local and global dynamics in galaxies. This is an
extension of some exploratory work currently underway. If successful there will
be a number of new avenues in galaxy dynamics, specifically related to the
interstellar medium evolution, to explore.
If you are interested in computational cosmology and galaxy formation,
but unsure about a specific research project, or you have general
questions about the research topics listed above, feel free to send me
an
email
(click here for address). Saint Mary's
actively encourages applications from students who wish to take time to
decide their research topic. Interested students are encouraged to
submit applications before February 2017, although later
applications will still be considered subject to the availability of
positions.
Below is a precis of my research interests, at a somewhat
technical level. Although slightly out-of-date now, it gives a
reasonable background to the research areas I am currently active in.
Formation of galaxies in hierarchical clustering cosmologies
Developing a detailed understanding of galaxy formation is one of the
primary goals of modern cosmology. The basic theory of baryons clustering
in a hierarchical system of dark matter halos was laid out by White &
Rees (1978,
MNRAS, 183, 341). In a hierarchical cosmology the
cooling time for baryons in halos is much shorter than the
free-fall collapse time, which results in star formation being
precipitated in
cool gas in low mass halos at very early epochs -- the `cooling
catastrophe' (White &
Frenk 1991, ApJ, 379, 52).
Simulations confirm this prediction and introduce a further problem:
the dense gas cores lose much of their
angular-momentum to the dark matter via dynamical friction (Navarro &
Benz 1991, ApJ, 380, 320;
Katz & Gunn 1991, ApJ, 377, 365).
The solution to both these problems is believed to be the inclusion of
feedback from supernovae (SN) and stellar winds. White & Frenk (1991)
have shown that, under reasonable assumptions, the energy input from SN
can be sufficient overcome the cooling catastrophe. However, it has
proven extremely difficult to implement feedback in hydrodynamic
simulations as it is a sub-resolution process. As part of my Ph.D.
thesis, I conducted a number of simulations comparing different ways of
returning feedback energy to the local gas in Smoothed Particle
Hydrodynamic (SPH) simulations of galaxy formation. My results have shown
that because the first generation of objects overcool (they must do so
before star formation begins), it is almost impossible to circumvent the
overcooling phenomenon without drastic feedback (Thacker & Couchman 2001,
ApJ, 545, 728). However the low resolution used in these studies (4000
(baryonic) particles per $L^*$ galaxy) and the complex interaction between
resolution and feedback rendered this conclusion preliminary. Note that
Binney et al (2001, MNRAS, 321, 471) indirectly support this conclusion.
My investigations of star formation within the galaxy formation process
show that the best method for preventing drastic (unphysical) radiative
losses following a feedback event is to use a brief period of adiabatic
expansion. In simulations with 40,000 particles per galaxy (Thacker &
Couchman 2001, ApJ, 555, L17, see also Science, July 6, 2001), I
have
found feedback can lead to a galaxy preserving 40% of its specific
angular momentum, which is an improvement over the 10% value typically
found in simulations (eg Navarro, Frenk & White 1995, MNRAS, 275, 56),
but remains below the value required to match the Tully-Fisher relation
for observed galaxies (Navarro & Steinmetz 2000, ApJ, 528, 607). However,
the scale length of the resultant galaxy is within 10% of that predicted
on theoretical grounds (Mo et al 1998, MNRAS, 295, 319).
Interaction of galaxies with the inter-galactic Medium
(IGM)
In the past two years, driven by an influx of new observations,
the study of galactic outflows has moved from the fringes of interest to
being a ``hot topic''. In collaboration with Scannapieco (UCSB), I have
developed an
n-body implementation of his semi-analytic galactic wind/outflow model
(Scannapieco & Broadhurst 2001, ApJ, 549, 28). We have confirmed a number
of conclusions from the semi-analytic work (Scannapieco, Thacker & Davis,
2001, ApJ, 557, 605), the most important being that outflows can strongly
suppress galaxy formation on 109 solar mass scales, thereby
solving the `missing satellites' problem for CDM. We have recently updated
the model to include metal enrichment (Thacker, Scannapieco & Davis,
2002, ApJ, 581, 836). With my colleagues, I have shown that simple,
plausible, assumptions for metal production can reproduce volume filling
factors and enrichment levels consistent with estimates from analytic and
semi-analytic models (Pei et al 1999, ApJ, 522, 604; Scannapieco et al
2002, ApJ, 574, 590). Future plans for these models include updating the
early universe physics to include cooling from molecular hydrogen.
In an effort to constrain these models further, specifically metal
enrichment
in the IGM, I recently became part of a N. America-Euro collaboration
(headed by Petitjean, CNRS) that has been analyzing quasar spectra
obtained by the UVES spectrograph on the European Southern Observatory
Very Large Telescope (VLT). The quasar spectra show absorption features of
CIV, SiIV and MgII which we have compared to
mock
spectra produced from
one of the highest resolution simulations of large-scale structure in the
IGM. Remarkably, indicating that we do not yet fully understand the
details of the enrichment process, the correlation function of CIV
systems
at z~3 suggests that enrichment patterns produced are dominated by
large systems which expell metals over very large ($>2$ Mpc comoving)
radii (Scannapieco et al, 2004, in prep). This is contrary to initial
ideas of comparatively uniform enrichment produced by dwarf systems.
Gravitational theory and simulations of large-scale
structure
The spectacular progress in uncovering the large-scale structure of our
universe over the past ten years has eclipsed growth in our understanding
of the non-linear evolution of structure formation. Almost 30 years ago
Peebles (1974, ApJ, 189, L51) postulated the `stable clustering
hypothesis' (SCH) which states that, in the highly non-linear regime,
small-scale gravitational clustering should exactly counterbalance Hubble
expansion. The SCH is important because it underlies a number of methods
for determining the non-linear evolution of the correlation function of
galaxies (eg Hamilton et al 1991, ApJ, 374, L1). To date, there have been
arguments suggesting that the SCH is valid (eg Jing 2001, ApJ, 550, 125),
while others argue that it fails (eg Smith et al, 2003, MNRAS, 341,
1311). The primary disagreement stems from the different methods of
determining whether the SCH holds; one can use pair-wise velocity
statistics, or the correlation function/power spectrum. The problem is
further complicated by the fact that while the SCH may not be exact for
individual halos, it could still be true in a statistical sense. In work
funded by the Pittsburgh Supercomputing Centre, conducted in collaboration
with Sheth, Colberg (Pittsburgh) and Couchman, we are currently conducting
simulations with sufficient resolution to test the SCH using both pairwise
and ensemble methods.
In support of this research, I have also been examining how to create very
precise initial conditions for simulations. On `glass-like' initial
conditions, standard interpolation methods fail to accurately reproduce
the
power spectrum near the Nyquist frequency. In collaboration with Couchman
and Scoccimarro (NYU), we are working on creating initial conditions that
use 2nd order Lagrangian perturbation theory to calculate the velocities
of particles (see Scoccimarro, 1998, MNRAS, 299, 1097) rather than the
usual first order method. This has particular relevance for higher order statistics, such as
skew and kurtosis, where first order methods are known to produce
inaccuracies unless the simulation is begun from extremely early epochs.
We plan to publically release a parallel implementation of the algorithm.
Programming and load balancing N-body codes on parallel
computers
The majority of my research on parallel codes has focused on shared
memory hardware (Thacker, Couchman & Pearce, 1998, in ``HPCS1998'',
Kluwer Academic). The simple
programming model afforded by shared memory has helped alleviate a number
of problems stemming from the complexity of the adaptive data structures
used in the HYDRA code. I have spent a significant amount of
effort in improving the parallel efficiency, primarily by parallelizing a
number of previously serial parts of the code, but also by improving the
data locality (and thus achieving better cache performance on RISC
processors).
Over the past year the focus of my work has been the completion
of a distributed memory (MPI-2) version of the HYDRA code (see
Thacker et al 2003, in ``HPCS2003'', NRC Research Press). This research is
conducted in collaboration with the Virgo Computational Cosmology
Consortium, and is the culmination of a three year effort. I first used
the code as part of the project examining the distribution of metals in
the IGM. For this project we conducted a hydrodynamic simulation with 65
million particles, using 64-128 CPUs of the SHARCNET facilities at
McMaster. The code is an exceptional piece of technology as it uses a
number of novel load-balancing techniques. Parallel scaling tests
conducted
at Lawrence Berkeley Lab on their IBM SP-3 have shown almost
perfect scaling out to 512 CPUs, and there is no reason not to
expect good performance for several thousand processors. Also, due to the
early release nature of MPI-2, it has proven particularly challenging to
implement the code efficiently on different platforms. I have had to work
closely with compiler engineers both to update support of the MPI-2
standard and also to improve performance.
Dr. Rob Thacker
319E Atrium Building
Department of Astronomy and Physics
Saint Mary's University
Halifax, Nova Scotia
Canada B3H 3C3
Electronic-mail: (click here for address)
Telephone: 902-420-5636
Facsimile: 902-496-8218
|
|
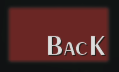 |
|